Standard Club has merged with North to form NorthStandard. Find out more about NorthStandard here or continue on this site to access industry news, publications and expertise, as well as club rules and contacts.
輸送手段における脱炭素化:コンプライアンスを達成するという選択
最近の科学報告書によると、人間の活動は前例のない、時には不可逆的な方法で気候を変化させています-つまり、気象パターンが変化し、海面が上昇し、極端な異常気象がより頻繁で激しくなってきています。
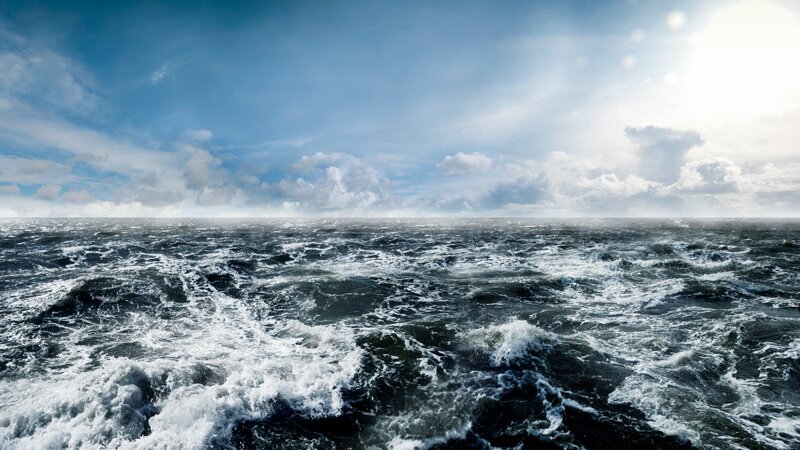
最近の科学報告書によると、人間の活動は前例のない、時には不可逆的な方法で気候を変化させています-つまり、気象パターンが変化し、海面が上昇し、極端な異常気象がより頻繁で激しくなってきています。国連(UN)は、この報告書の調査結果を「人類へのコード・レッド(厳戒警報)」と述べました。
国連の専門機関として、国際海事機関(IMO)は気候変動とその影響と闘う取り組みへの貢献を約束しました。
2021年6月に開催されたIMO 第 76 回会合海洋環境保護委員会(MEPC 76)で、温室効果ガス(GHG)排出量削減に向けた必須の目標に基づいた技術対策および運航対策の提供に多くの時間が費やされました。これらの改正案は2022年11月1日に発効し、2023年1月1日から適用されます。
この目標に基いたアプローチにより、船主と運航会社は、以下の技術対策および運航対策を組み合わせることにより、年間炭素強度削減係数を達成することができます。
-
- 既存船エネルギー効率指標(EEXI)の計算と検証、
- 運用中の燃費実績(CII)格付け制度に関連する評価メカニズム(AからEの五段階評価)の導入、および
- 船舶エネルギー効率管理計画書(SEEMP)の使用と監査の強化。
船舶用脱炭素ソリューション
クラブの 過去の記事で強調されているように、新造船へのエネルギー効率評価指標(EEDI)要件(1)が適用されてからしばらく経ちます。
2022年11月1日から、既存船舶は、到達既存船エネルギー効率指標(EEXI)を計算し、それが規制値以下であるかどうかを判断する必要があります。一方、EEDI認定船舶は、EEDI到達値が規制値以下であるかどうかを判断する必要があります。
EEXI/EEDI到達値が規制値以下である船舶は、EEXIテクニカルファイル(EEXI到達値の計算に使用されるデータを含む)を作成し、国際エネルギー効率証書(IEEC)発行を旗国政府に申請する必要があります。ただし、EEXI/EEDI到達値が規定値を超える場合は、EEXI到達値を改善する措置を講じる必要があります。
エネルギー効率の高い技術(EET)の改造や低炭素/カーボンニュートラル燃料への転換に加え、EEXI規制では、機関出力制限(EPL)とシャフト出力制限(SHaPoLi)の両方を含む優先された出力制限を使用でき、動作中のエンジン負荷と燃料消費を制限します。このソリューションは比較的非侵襲的であり、資本コストが低く、ほとんどの船種に適用できます。 ただし、その後のCII評価に影響を与える能力は制限されます。
国際クリーン交通委員会(International Council on Clean Transportation: ICCT)による2020年の 調査 では 、次のように述べています。「30%未満のEPLシナリオでは、2018年船舶からのCO2排出量削減はごくわずかですが、30%EPLはコンテナ船と石油タンカーでCO2排出量を2%削減、ばら積み貨物船で3%削減すると推定されています。より大きな50%以上EPLは、船種と規模に応じて、船舶のCO2排出量を8%から19%削減する可能性があります。さらに重要なことに、船体売上高の伸びにより新しい船舶にEPLが必要とされない場合、利益は時間とともに減少します。」
EEXI規制の枠組み
さらに、メンバーは、運航炭素強度削減に同時に取り組むためのステップを検討する必要があります。CIIは船舶運航方法に基いているため、その価値は次の方法で改善できます。
- 減速または減速航海(スロースティーミング)での運航、
- ウェザールーティング(最適航路選定)、
- 貨物積載量を減らす、
- 船舶の一般的な状態を改善する(すなわち、船内洗浄とコーティング)、
- 低炭素またはカーボンニュートラル燃料への切り替え、
- 船体空気潤滑システム、風力補助などのエネルギー効率の高い技術の導入。
代替燃料と新興技術の比較
代替燃料と新技術に関しては、万能の解決策はなく、船種/船齢、商圏、改造費用、運航予算、燃料価格/可用性、インフラ設備の開発、安全性の問題などに基づいて適切な選択肢を選択する際に多くの考慮事項があります。
LNG、LPG、バイオ燃料、メタノール、アンモニア、水素、および燃料電池とバッテリーの使用は、主な選択肢の一部です。それぞれに長所と短所があり切り替えるのは簡単ではありません。エンジンやその他の機械は、対象となる燃料を燃焼することができなくてはいけません。
様々な燃料選択肢に関連する考慮事項は他にも多くあります。たとえば、バイオ燃料は、酸化安定性、コールドフロー特性、微生物増殖のリスク、フィルターの目詰まり、エンジンデポジットの増加など、いくつかの技術的課題をもたらし、従って慎重な取り扱いが必要です。
船舶での他の代替燃料選択肢の取り扱いも複雑になる可能性があり、高度な訓練を受けた乗組員が必要になります。ほとんどの液化ガスは極低温での保管を要し、特定の安全基準を満たす必要があります。たとえば、水素は可燃範囲が広く、アンモニアは非常に毒性があります。乗組員をこれらに対する接触から守るためには、厳格な対策が必要になります。
水素はクリーンな燃料ではありますが、水素燃料の製造はエネルギーを大量に消費し、炭素副産物が発生します。現在ブラウン水素と呼ばれているものは、褐炭からガス化によって生成されます。天然ガスからグレー水素を生成するプロセスは、二酸化炭素排出を伴います。ブルー水素は、グレー水素生産で発生する温室効果ガスの二酸化炭素を回収して貯蔵します。究極のクリーンな水素資源であるグリーン水素生産は、再生可能エネルギーを使用して水素燃料を生成しますが、これは非常に高額になる可能性があります。
同様に、グリーンアンモニアは、従来のアンモニアの2〜4倍のコストがかかります。グリーンおよびブルーアンモニアバリューチェーン(価値連鎖)は、使用する水素製造方法が異なります。グリーンアンモニアは水の電気分解から生成され、ブルーアンモニアは天然ガスを使用して従来の経路から生成されますが炭素回収が加わります。
貯蔵容量の観点から、燃料のエネルギー密度/燃焼量は重要です。燃料のエネルギー密度が少なくとも現在のエネルギー密度に匹敵しない場合は、船上により多くの貯蔵スペースが必要になります。たとえば、水素、アンモニア、およびメタノールはエネルギー密度が低いため、船内でより大きなタンクが必要になります。
燃料が広く普及されるようになるには、十分な拡張性が必要です。つまり、インフラストラクチャと需要の両方が存在する必要があります。これは通常の定期航路の船舶にとっては簡単かもしれませんが、港湾間を運航する船舶は相対的に少ない選択肢を見つけるのに苦労するでしょう。
ほとんどの代替燃料と技術は、まだ技術的、経済的および環境的な面における監査を受けています。それでも、ここでは代替燃料の商業的および運航上の実行可能性を評価するために、利用可能な選択肢の長所と短所のいくつかを以下に示します。
代替燃料 | 長所 | 短所 |
液化天然ガス(LNG) |
|
|
液化石油ガス(LPG) |
|
|
バイオ燃料 |
|
|
水素(H2) |
|
|
アンモニア(NH3) |
|
|
メタノール(CH3OH) |
|
|
エネルギー効率化技術(EETs) | |
船体形状の最適化 | 船体形状の最適化は、水と船体の間の造波抵抗と摩擦抵抗を最小限に抑えることに焦点を当てています。摩擦抵抗が減少すると、特に速度が低下したときに、船のエネルギー効率が向上します。船体を流体力学的に最適化する方法は次のとおりです。
最適化対策は通常、新造船に適用されますが、既存の船体の改造にも適用されます。ただし、設計変更の検討前に、船体の性能と運航プロファイルを詳細に理解することが重要です。その場合、通常は総合的な一連の模型試験と船舶の流れシミュレーション(CFD)評価が必要です。船体形状最適化を検討する場合、CFD解析に姉妹船を含めて、フリートのコストを削減することが有益です。 |
船体コーティング | 摩擦抵抗を和らげる1つの方法は、汚れを落とすコーティングによって船体の滑らかに保つことです。近年、コーティング技術には多くの発展が見られています。たとえば、コーティング表面に顕微鏡でしか見えない微細な水層を閉じ込めて船体周りの水の流れを滑らかにする物質であるハイドロゲルの導入などです。 ハイドロゲルを含むコーティングを使用すると、船体の表面が微視的なレベルで液体のようなはたらきを見せます。ただ単に船体汚損が付着を防ぐだけでなく、船体の摩擦を大幅に減らすことにつながるのです。 |
空気潤滑 | 空気潤滑は、船体と海水の間に気泡の層を作ることで船体の摩擦抵抗を減少させる方法です。 この摩擦削減により、省エネ効果が生まれ、船舶の燃料消費量が削減されます。自動システムにより、速度に応じてコンプレッサー/ブロワーを調整します。理想的な状況では、空気噴射システムは大幅な燃料節約に加え、CO2排出量の最大15%の削減を達成できます。 このシステムは、V字型の船体底部を有する船舶と比較すると、V字型の船体底部の空気は平底よりもはるかに簡単に潤滑するため平底においてより効果的です。 |
プロペラと舵 | 可能な限りプロペラ回転流を回収することで船舶のエネルギー効率を改善するため、またはプロペラへの流入とプロペラの中および後方に流入する回転流を整流することで最高のパフォーマンスを得るために多くの装置が設計されています。 |
電気(電池式)推進 | 近年、リチウムイオン(Li-ion)バッテリーを使用したバッテリーのみの電気推進が、小型の近海船にうまく適用されています。 ハイブリッドシステムで2ストローク機関と組み合わせたバッテリーの可能性も、より大型の外航船に向けて調査されています。 |
ハイドロゲン燃料電池 | ハイドロゲン燃料電池は、電池と同じように機能します。つまり、電解質膜を通過する帯電した水素イオンの動きを使用して化学エネルギーを電気エネルギーに交換し電流を生成します。そこで酸素と再結合させて水を生成します。これが熱風とともに燃料電池の唯一の排出物です。バッテリーほど消耗せず充電する必要もなく、電力密度が高く重量も軽くなっています。 ただし、高価であり、漏えいが適切に処理されない場合、水素ガスが蓄積されて爆発につながる可能性があります。したがって、水素の貯蔵場所と燃料電池キャビンには、安全な整合性レベルを確保する適切な対策が必要です。 |
Shore to Ship(陸側施設から燃料供給を受ける作業)方式(Cold Ironing) | これは、舶用主機と補助エンジンがオフになっているときに、停泊中の船舶に岸壁から電力供給するプロセスです。船舶が入港する場合、補助エンジン(発電機)は通常、貨物の運航、非常用機器、冷房、暖房、照明、および船内使用に必要な電力を供給するために使用されます。発電機の電源を切り、港湾の電源補給所に接続するだけで、燃料消費量を節約し、その後、騒音と大気排出量を削減できます。 電力の必要が低い小型船舶は、通常のグリッド電圧と周波数を利用できます。ただし、必要電力が高い大型船の場合、この機能を提供できるのは限られた港湾のみである可能性があります。 |
廃熱回収システム | 主機排熱回収システム(WHRS)は、排気ガスから排熱を回収して電気エネルギーに変換し、余熱はさらに船舶への供給(温水や蒸気など)に使用できます。このシステムは、排熱回収ガスボイラー(または石油焚きボイラーと組み合わせて)、パワータービンおよび/またはオルタネーター付き蒸気タービンで構成することができます。船舶のレイアウトを再設計することにより、船舶ボイラーを効率的にこれらのシステムにより適合させることができます。舶用主機の燃料消費量は3%から8%削減される可能性があり、全体的な排出削減に貢献します。 廃熱回収は船内で十分に可能であると証明されていますが、船内のエンジンの規模、数、使用法、効率により、その可能性は変動する可能性があります。さらに、これらの対策は、再設計、鉄骨工事、余剰重量などに関連する多大なコストと労力がかかるため、通常、改造には適していません。 |
炭素の回収と保管 | この技術は、船舶開発においてはまだかなり初期段階にあります。これには、船舶の排気ガスからの炭素排出物の隔離および回収を通して、大気中への放出を防ぐことが含まれます。ただし、船舶が港湾に到着するまで液体貨物を収集するには適切な極低温貯蔵タンクが必要です。その後、炭素は地層の地下に恒久的に貯留されるか、炭素消費産業で利用されます。 |
ソーラーパネル | ソーラーパネルは、太陽光を電気に変換する発電方式です。船舶のソーラーパネルは現在あまり一般的ではありませんが、自動車運搬船、バルカー、旅客フェリー、小型国内船など、ここ数年、特定の種類の船舶に船舶用ソーラーパネルの設置が行われています。このソリューションは、スペースを必要とするためコンテナ船には適さない場合があります。 このテクノロジーはまだ初期段階にあり、時間の経過とともに安価になると予想されますが、パネル効率が大幅に向上したり、消費スペースが少なくなる可能性はほとんどありません。 |
風力推進システム | 風力補助推進システム(帆、凧、固定翼、フレットナーローターなど)は、昔ながらのコンセプトに近代化された機能を織り交ぜています。IMOはこの技術を認識しており、 MEPC.1/Circ. 815に風力推進の効果を組み込んでいます。ただし、これは一次推進システムを補強する補助推進システムと見なされます。実際、ほとんどの風力補助推進システムは、操作するために二次エネルギー供給源を必要とします。例えば、フレットナーローターは、空力推力を発生させるためにモーターによって始動される必要があります。 このようなシステムの場合、利用できる風が発生していること、したがって風力補助船舶の運用エリアが最も重要な要素です。燃料節約の可能性に加えて、運用コスト(メンテナンス、スペアパーツ、コンポーネント交換など)を考慮することが重要です。 |
結論
脱炭素化への道は、燃料オプション、技術の進歩、運用の改善に関する考慮事項を含みます。どのオプションが安全かつ拡張可能で、商業的に実行可能なソリューションを提供する可能性があるかを理解する必要があります。利用可能なすべてのオプション、および特定の船舶と運航に対する適合性を考慮することは、業界全体の脱炭素化に向けて進む際に、海運業界をより安全に維持するための鍵となります。
変更には時間がかかるものだと推測しがちですが、これは間違いです。船舶の運航方法や燃料供給方法は既に変化を見せています。今後の課題の規模、進化する市場状況、規制状況を考えると、これらの問題を今すぐ慎重に検討し始めることが不可欠です。
どのオプションを選択するかに関係なく、代替燃料は排出削減を達成するという最終目標において重要な役割を果たします。どの燃料オプションが最適かを判断するのは難しい場合があります。この窮状を踏まえて、クラブは海運輸送における脱炭素化に関する多くの側面を検討するワーキンググループと諮問委員会を結成しました。これは、メンバーが今後の規制に備え、これらの課題に対処するのを支援することを目的としています。
(1)IMO RESOLUTION MEPC.203(62)に従い、「新造船」とは以下の船舶を意味します。
-
- 2013年1月1日以降に造船契約書が締結されたもの。または
- 造船契約書がない場合、2013年7月1日以降にキールが敷設されている、もしくは同様の造船段階にある。または
- 納入は2015年7月1日以降である。
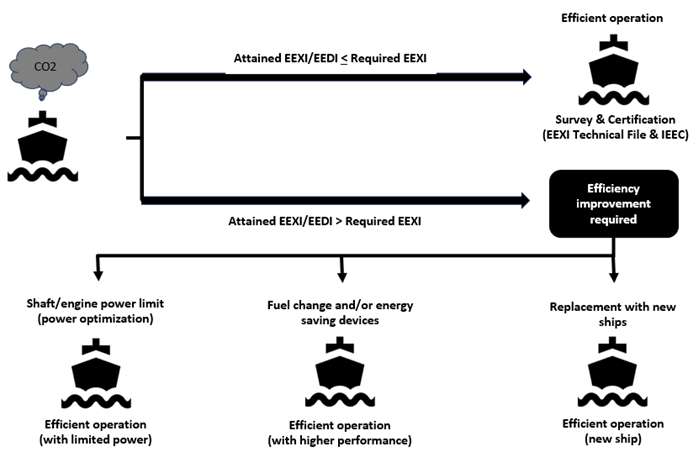
Additionally, members will need to consider steps to address operational carbon intensity reduction simultaneously. As CII is based on how a ship is operated, its value can be improved by:
- operating at a reduced speed or slow steaming;
- weather routing;
- reducing cargo intake;
- improving general condition of the ship (i.e., hull cleaning & coating);
- switching to a low-carbon or carbon neutral fuel;
- installing energy efficient technology, like hull air lubrication systems, wind assistance, etc.
Comparison of alternative fuels and emerging technologies
In terms of the alternative fuels and emerging technologies, there is no one-size-fits-all solution, and a lot of considerations will go into selecting appropriate option based on ship type/age, trading area, retrofitting costs, operating budget, fuel price/availability, infrastructure development, safety issues, etc.
LNG, LPG, biofuel, methanol, ammonia, and hydrogen, as well as the usage of fuel cells and batteries, are some of the main options. Each has its own set of advantages and disadvantages and switching between them is not easy - engines and other machinery will need to be capable of burning the fuel in question.
There are numerous other considerations in relation to the various fuel options. For example, biofuel brings a handful of technical challenges such as oxidation stability, cold flow properties, risk of microbial growth, clogging of filters, and increased engine deposits; and hence, it requires careful handling.
Handling of other alternative fuel options on vessels could be complex as well and will require a highly trained crew. Most of the gases in liquid form requires storage at cryogenic temperature - specific safety standards will need to be satisfied. Hydrogen, for example, has a wide flammability range, while ammonia is highly toxic. Stringent measures will be required to protect crew from exposure.
Hydrogen is a clean fuel; however, manufacturing hydrogen fuel is energy-intensive and has carbon by-products. What is now called brown hydrogen is created through coal gasification. The process for producing grey hydrogen from natural gas throws off carbon waste. Blue hydrogen uses carbon capture and storage for the greenhouse gases produced in the creation of grey hydrogen. Green hydrogen production – the ultimate clean hydrogen resource – uses renewable energy to create hydrogen fuel, which could be quite expensive.
Similarly, green ammonia will cost two to four times as much to make as conventional ammonia. The green and blue ammonia value chains differ in the hydrogen production method used; green ammonia being generated from water electrolysis and blue ammonia being generated from a conventional pathway, using natural gas, but with the addition of carbon capture.
In terms of storage capacity, energy density/calorific value of the fuel is critical. More storage space on the ship will be required if a fuel does not have an energy density that is at least comparable to what it has now. Hydrogen, ammonia, and methanol, for example, have a lower density, requiring larger tanks onboard ships.
For a fuel to become widely used, it must have adequate scalability, i.e., both the infrastructure and the demand must be there. This may be easier for ships on regular liner route, but ships traveling between ports will have a difficult time finding the relatively scarce option.
Most of the alternative fuels and technologies are still undergoing technical, economic, and environmental review. However, in order to evaluate the commercial and operational viability of alternative fuels, we have listed below some of the pros and cons of the options available.
Alternative fuels | Advantages | Disadvantages |
Liquified Natural Gas (LNG) |
|
|
Liquified Petroleum Gas (LPG) |
|
|
Biofuels |
|
|
Hydrogen (H2) |
|
|
Ammonia (NH3) |
|
|
Methanol (CH3OH) |
|
|
Energy efficiency technologies (EETs) | |
Hull form optimisation | Hull optimisation focuses on minimising the wave resistance and friction between water and hull. The reduced frictional resistance increases energy efficiency of the ship, particularly at reduced speeds. Following are the ways by which hull hydrodynamic performance can be improved:
Optimisation measures are generally applied on new-built ships but also applicable to retrofitting of existing ships. However, it is important to understand in detail the ship’s performance and its operating profile before considering any design modification. Usually, a comprehensive series of model tests and computational fluid dynamic (CFD) assessments are required in such cases. When considering hull form optimization, it is beneficial to include sister ships in the CFD analysis to reduce the cost for the fleet. |
Hull coatings | One way of lowering the frictional resistance is to improve the smoothness of a hull by means of coatings that reduce fouling. In recent years there has been a lot of development in the coating technology, e.g. introduction of hydrogel, a component that traps a microscopic layer of water on the coating’s surface, smoothing the water flow around the hull. The use of hydrogel containing coatings makes the surface of the hull behave like a liquid on a microscopic level. This not only deters the fouling from settling in the first place, but also significantly reduces hull friction. |
Air lubrication | Air lubrication is a method to reduce the frictional resistance between the ship’s hull and water using a sheet of air or air bubbles. This reduction creates energy-saving effect and cuts down on the fuel consumption of the ship. An automation system regulates the compressors/blowers depending on speed. In ideal situations, an air injection system can achieve up to 15% in the reduction of CO2 emissions together with significant fuel savings. As compared to ships with V-shaped bottoms, this system is more effective on flat bottoms as because the air on a V-shaped bottom will flow away much more easily than a flat bottom. |
Propellers and Rudders | Numerous devices have been designed for improving the ship’s energy efficiency by recovering as much as possible of the rotational energy in the flow from the propeller, or to provide some pre-or post-rotation of the in-flow into and after the propeller to ensure best performance. |
Electric (battery powered) propulsion | In recent years, pure battery-electric propulsion, using Lithium Ion (Li-ion) batteries, has been successfully applied on small, short-sea vessels. The potential for batteries in combination with a two-stroke main engine in a hybrid system is being evaluated for larger ocean-going vessels. |
Hydrogen fuel cells | Hydrogen fuel cells works in a similar manner to an electric battery, i.e., it converts chemical energy into electrical energy using the movement of charged hydrogen ions across an electrolyte membrane to generate current. There they recombine with oxygen to produce water – a fuel cell’s only emission, alongside hot air. It does not deplete or need charging and has a higher power density and lower weight than batteries. However, it is expensive and any leakage, if not handled properly, may cause hydrogen accumulation and explosion. Therefore, the hydrogen storage place and fuel cell cabin require appropriate measures to ensure safe integrity levels. |
Shore to ship power (cold ironing) | This is the process of providing shoreside electrical power to a ship at berth while its main and auxiliary engines are turned off. When a ship is in port, auxiliary engines (generators) are commonly used to provide required power for cargo operations, emergency equipment, cooling, heating, lighting as well as domestic use. By simply turning off generators and plugging in to an electrical supply point in the ports, fuel consumption saving and subsequently reduction of noise and air emission can be achieved. Smaller vessels with low power requirements can make use of normal grid voltage and frequency. However, for larger vessels with high power requirements only limited ports may be able to provide this facility. |
Waste heat recovery systems | A waste-heat recovery system (WHRS) recovers the thermal energy from the exhaust gas and convert it into electrical energy, while the residual heat can further be used for ship services (such as hot water and steam). The system can consist of an exhaust gas boiler (or combined with oil fired boiler), a power turbine and/or a steam turbine with alternator. Redesigning the ship layout can efficiently accommodate the boilers on the ship to better fit these systems. There is a potential for a reduction in main engine fuel consumption estimated at 3% to 8% which contributes to emissions reductions overall. Waste heat recovery is well proven onboard ships, but the potential can be variable depending on the size, numbers, usage and efficiency of the engines on board. Furthermore, these measures are usually not relevant for retrofitting, due to large costs and efforts related to redesign, steel work, extra weight, etc. |
Carbon capture and storage | This technology is at very early development stages for ships. It involves isolation and capturing carbon emissions from the ship’s exhaust and preventing them from entering the atmosphere. However suitable cryogenic storage tanks are needed to collect liquid cargo until the ship reaches port. Thereafter, the carbon can either be stored permanently under the ground in geological formations or utilised in carbon-consuming industries. |
Solar panels | Solar panels are devices that convert light from the sun into electricity. Solar panels on ships are not very common at present, but some installations have been done over the last years on certain types of ships including car carriers, bulkers, passenger ferries and smaller domestic vessels by using marine grade solar panels. This solution may not suit container vessels because of the space required. This technology is in its infancy and is expected to become less expensive over time, but the panels are unlikely to become much more efficient or less space consuming. |
Wind assisted propulsion systems | Wind-assisted propulsion systems (e.g., sail, kite, fixed-wing, Flettner rotors) utilises an old concept with a modern edge. The IMO has recognized this technology and included the effects of wind propulsion in MEPC.1/Circ. 815. However, it is considered as an auxiliary propulsion system that augments the primary propulsion system. In fact, most wind-assisted propulsion systems require a secondary source of energy to be operated, e.g. Flettner rotors need to be started up by motors to develop their aerodynamic thrust forces. For such systems, the availability of wind and therefore the operation area of wind-assisted vessels is the most relevant factor. It is vital to consider operational costs (maintenance, spare parts, replacement of components, etc.) in addition to the fuel saving potential. |
Conclusion
The route to decarbonization involves consideration regarding fuel options, technology advancements and operational improvements. It is necessary to understand which option has the potential to deliver safe, scalable, and commercially viable solution. Considering all available options, and their suitability for specific vessels and operations, is key to ensuring a safer shipping industry as we move towards industry‑wide decarbonisation.
While it may be tempting to assume the timescale of any change is long, this would be a mistake. The way ships are operated and fuelled is already changing. Given the scale of upcoming challenges, evolving market conditions and regulatory landscape, it is imperative to start giving these issues a careful thought now.
Regardless of which option is chosen, alternative fuels will play a key role in the ultimate goal of achieving emissions reduction. Determining which of the fuel options is best suited can prove challenging. Understanding this predicament, the club has formed a working group and advisory panel that looks at many aspects around decarbonisation in shipping. This is aimed to assist members to prepare for the upcoming regulations and meet these challenges.
(1)As per IMO RESOLUTION MEPC.203(62), 'new ship' means a ship:
for which the building contract is placed on or after 1 January 2013; or
in the absence of a building contract, the keel of which is laid or which is at a similar stage of construction on or after 1 July 2013; or
the delivery of which is on or after 1 July 2015.
カテゴリー: Alternative Fuels